We found this amazing article about the effects of glyphosate on the gut microbiome and health in general on the Weston A Price Foundation website. Written by Stephanie Seneff PHD in 2021, we felt it was so important that we wanted to share the information here.
The full article and all the scientific references can be found on the Weston A. Price Foundation website.
ARTICLE SUMMARY
- The gut microbiome is a symbiotic collection of trillions of bacteria, viruses and fungi that produce many biologically useful molecules, notably B vitamins, which host cells are incompetent to synthesize on their own.
- The gut and the brain are in close communication via the “gut-brain axis.” Many modern neurological and other diseases appear to have their origins in the gut, including autism, depression, multiple sclerosis, Parkinson’s disease and Alzheimer’s.
- Animal studies of gastrointestinal dysfunction have produced findings consistent with chronic glyphosate poisoning. Glyphosate likely also plays a role in the modern epidemic of constipation, by essentially paralyzing the gut.
- Research indicates that lactobacillus and bifidobacteria are the classes of gut bacteria that seem to be most sensitive to glyphosate. Studies of infants point to a dramatic reduction of bifidobacteria species in the infant gut in recent decades. Glyphosate is a plausible causal factor explaining this loss.
- An imbalance and/or deficiency in three short-chain fatty acids—acetate, propionate and butyrate—has broad consequences not only on gut health but also on brain health. Glyphosate raises the gut pH, likely reducing the amount of butyrate produced in the gut (which depends on a low gut pH). The best natural source of butyrate is organic butter from grass-fed cows.
The gut microbiome is a teeming collection of trillions of bacteria, viruses and fungi, which have made the human gut their home. They are dominated by two phylogenetic groups (Bacteroidetes and Firmicutes). Their relationship with the host is symbiotic rather than parasitic, because they perform many functions for the host, producing all sorts of biologically useful molecules that the host cells are incompetent to synthesize on their own.
There are estimated to be 1014 microbes residing in the human gut. While it has been widely claimed that they outnumber our own cells by a factor of ten, careful analysis suggests that they may “only” match our own cells one-to-one.1 Nonetheless, it is undisputed that their collective genome carries more DNA code (information) than ours by a factor of at least one hundred.2
A genomic assessment of the human microbiota demonstrates that they collaborate symbiotically to produce thiamin (B1), riboflavin (B2), niacin (B3), pantothenate (B5), pyridoxine (B6), biotin (B7), folate (B9) and cobalamin (B12), and that these B vitamins can significantly augment vitamins supplied from food. Human cells are incompetent to produce any of these vitamins. Collectively, the microbial colonies contain a large number of enzymes that specialize in various steps in the synthesis of these essential nutrients.3
For most of the last century, the gut microbiome was pretty much ignored in research on the human body. This is probably due to the fact that it was functioning well; therefore, we did not notice all the things the microbes were doing for us. In recent years, however, the number of papers on the gut microbiome has exploded. Figure 1 shows a graph of the number of papers per one hundred thousand published in PubMed each year from 2010 to 2020, when the papers are retrieved on the search term “microbiome.”
While some researchers claim that animals cannot live without their gut microbes, modern-day experiments have shown that germ-free mice are able to survive, but they need to be supplied with much larger amounts of the B vitamins (B1, B6, B7, B9 and B12) to maintain good health.4 Bifidobacteria and lactobacillus are especially important for supplying these vitamins to the host.2
Surprisingly, germ-free mice have a much more acute stress response than mice raised in a natural environment. Researchers found that “restraint stress” (a technique used to assess stress responses in animals) caused a markedly increased elevation of plasma adrenocorticotrophic hormone (ACTH) and corticosterone (indicators of stress) in germ-free mice. If the gut of these mice was reconstituted with Bifidobacterium infantis early on, this exaggerated response disappeared, but if this reconstitution was delayed to a later stage in development, it was no longer effective.5Thus, at least for mice, it is crucial for brain health that the infant acquire a healthy gut microbiome in the first few weeks of life. Commensal microbiota, particularly bifidobacteria, are essential for the postnatal development of an appropriate stress response in mice.
Researchers now widely acknowledge that the gut and the brain are in close communication via the “gut-brain axis,” and a lot of this communication involves signals released by the microbes in the gut.6 Communication happens via the lymph system, via the blood circulation and via the vagus nerve.7 Many modern diseases are now believed to have their origins in the gut, including autism, depression, multiple sclerosis, Parkinson’s disease, Alzheimer’s and others.8
A seminal paper published in 2016 revealed that gut microbes are essential for maintaining neurogenesis (the creation of neurons) in the hippocampus.9 Furthermore, the authors demonstrated that antibiotics can have a profound effect on the brain. Antibiotic treatment led to a reduced population of an immune cell type called monocytes in the hippocampus of mice, and this in turn caused a reduction in neurogenesis in the hippocampus, along with impaired cognitive function. However, the researchers found that the effects could be reversed through probiotic supplements and increased physical exercise.
Reading any one of the recent papers on the gut microbiome can be a seemingly overwhelming task. The complexity of the space is enormous, and each person’s microbiome is unique, with many species coinhabiting the gut in a symbiotic relationship with one another and with us, their host. Which species take hold depends in part on the microbiome of the mother at the time the child is born. If the birth is through C-section, then the microbes that take up residence in the child’s gut resemble more the species that normally inhabit the skin, rather than those that inhabit the gut. This can set the child up for a rough start in terms of achieving a proper homeostasis in the gut.
Breast milk is designed to help nourish the lactobacillus and bifidobacteria that normally thrive in the infant gut, feasting on the lactose and casein in the milk. When solid foods are introduced, the microbiome can change dramatically to accommodate the rich variety of nutrients in the newly introduced foods.
AUTISM AND THE GUT
Perhaps not surprisingly, breastfeeding is protective against autism. A sibling case-control study found an odds ratio for autism of 0.166 for children who were exclusively breastfed early in life.10Clinicians are now recommending prebiotics, probiotics and postbiotics as treatment programs for autism.7
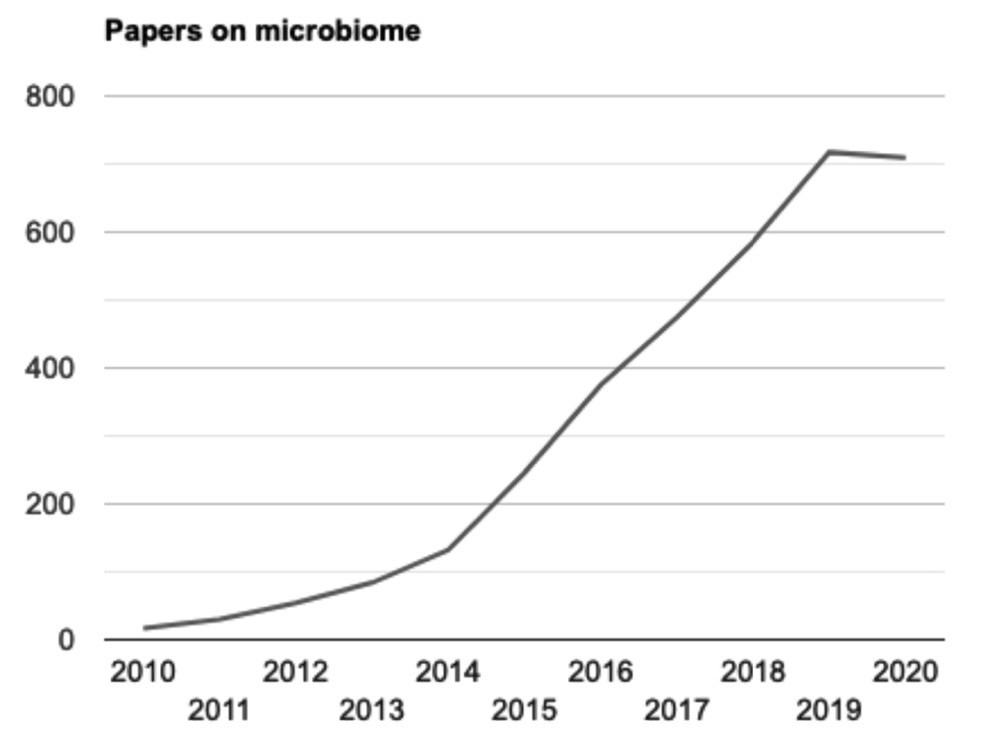
It is now indisputable that many autistic children suffer from gut problems and that this is almost surely a contributory factor to their autistic symptoms.7 An inflammatory gut and a leaky gut barrier allow pathogens and toxic microbial metabolites, such as lipopolysaccharide (LPS) and phenolic compounds such as p-cresol, to enter the general circulation, causing a systemic inflammatory response. Alterations in the gut microbiome also contribute to the disease process. Cytokines (inflammatory agents) released by immune cells activate the vagus nerve, altering activity levels in the central nervous system. Bacterial metabolites can breach the gut barrier and subsequently breach the blood-brain barrier, which is also impaired in association with autism. These then induce an inflammatory response in the brain, setting up a chronic low-grade encephalopathy which is associated with mood disorders and cognitive issues.
A remarkable study published by Hsiao and colleagues in 2013 involved inducing autism in the offspring of mice through a process termed maternal immune activation (MIA).11 They then studied the distribution of microbes in the gut of the autistic offspring and found overexpression of certain Clostridia species that were producing a metabolite called 4-ethylphenylsulfate (4EPS). This metabolite was forty-six-fold higher in the autistic mice than in controls. The researchers noted that it is very similar to p-cresol, which is known to be elevated in humans with autism.12Astonishingly, they found that they could produce anxiety in control mice simply by exposing them to 4EPS.
The demand placed on the liver to conjugate these microbially produced phenolic compounds with sulfate interferes with its ability to detoxify certain drugs and toxic metals such as mercury through sulfation. This was demonstrated directly in a study that showed that acetaminophen (Tylenol) was ineffectively sulfated by people with elevated urinary p-cresol sulfate. Sulfation is essential to detoxify acetaminophen, and its toxicity has been linked to autism.13,14
GUT BARRIER
The surface of the gastrointestinal tract is made up of a single layer of tightly interconnected epithelial cells. These cells are covered with mucus, a complex biological material that forms a barrier protecting the cells from damage by bacterial enzymes and toxins, and by extremes in the acid-base continuum. At the same time, it allows passage of gases, nutrients and many proteins.15
Mucus consists of highly sulfated “mucins,” which are made up of a peptide core to which are attached long chains of sulfated glycosaminoglycans (GAGs). The protective layer is highly viscous and well hydrated; that is, it is a layer of gelled water maintained by the sulfate anions. Under healthy conditions, it forms an excellent barrier that keeps the surface layer of cells safe from attack by any potentially damaging products in the intestinal lumen. The mucins in the colon are especially high in sulfate density, and this is thought to protect them from degradation by bacterial enzymes that can remove GAGs.16
THE LOSS OF BIFIDOBACTERIA
A seminal paper published in 2018 by Henrick and colleagues examined trends over time of the pH of the infant gut, going back one hundred years to the 1920s.17 They started by pointing out that the infant gut microbiome has “important long-term health implications.” The authors’ working hypothesis was that something in the modern environment was causing bifidobacteria to become much less able to dominate the infant gut microbiome, particularly in “resource-rich” countries. Whereas a paper published in 1913 described the infant gut as an “almost pure culture” of bifidobacteria,18 today’s studies find that the microbial mix in the infant gut is much more diverse. Another marker of the changes are the short polymers of simple sugars called human milk oligosaccharides (HMOs) contained in substantial amounts in human milk. Infants today excrete undigested HMOs in their feces in large amounts.19
When Henrick and co-authors plotted infant fecal pH reported in publications ranging in date from the early 1920s to the present, the plot revealed a striking contrast between publications prior to 1980 and those published after 1980.17 In fact, the data can be fully divided into two quadrants: all publications prior to 1980 showed a fecal pH that was less than 5.5, whereas all those published after 1980 gave a value greater than 5.5, with the highest values (up to 6.5) appearing after 2000. The authors proposed that the pH change was due to a dramatic reduction in bifidobacteria species in the gut in recent decades.
CAN GUT DISORDERS BE EXPLAINED BY GLYPHOSATE?
Glyphosate is the active ingredient in the pervasive herbicide Roundup. The United States, with 4 percent of the world’s population, consumes 20 percent of the world’s glyphosate. According to the Centers for Disease Control and Prevention (CDC), one in fifty-four children aged twelve years old is now on the autism spectrum. The United States has by far the most expensive health care costs among the industrialized world, yet we rank very poorly in infant mortality, maternal mortality and life expectancy.
Glyphosate was introduced into the food chain in 1974, and the genetically engineered Roundup-Ready crops started to become abundant in 2000. These include corn, soy, sugar beets, canola and alfalfa. Increasingly, glyphosate is also being sprayed as a desiccant or ripener just before harvest on several major crops, including wheat, oats, barley, lentils, garbanzo beans, chick peas, sugar cane, sunflower seeds and other oil seed crops. As shown in Figure 2, inflammatory bowel disease has been increasing in prevalence over time in lockstep with the rise in glyphosate usage on core crops.20
Glyphosate kills all plants except those that have been genetically engineered to resist it, and the primary mechanism is through suppression of EPSP synthase, an essential enzyme for the synthesis of aromatic amino acids (amino acids containing a ring structure). While it has been argued that humans are not sensitive to glyphosate because human cells don’t make EPSP synthase, this view does not take into account the gut microbiome. Researchers from Europe used a bioinformatics approach to predict the potential impact of glyphosate on gut microbes, on the basis of the specific class of the EPSP synthase enzyme they possess. Conservatively, they concluded that 54 percent of species in the core of the human gut microbiome possess the class I form of the enzyme that is sensitive to glyphosate.21
The alteration in the balance of gut microbes in infants today compared to the early 1900s fits well with the observed effects of glyphosate on gut microbes. A study on the effect of glyphosate on poultry microbiota showed that bifidobacteria were the most sensitive to glyphosate of all species studied.22 This is the species that used to be dominant in the gut of an infant consuming only breast milk. While Henrick and colleagues suggested that the increase in C-section deliveries, the increased use of antibiotics and/or the increased practice of formula feeding might account for the loss of bifidobacteria,17 glyphosate’s direct harm to bifidobacteria is a plausible causal factor. Zen Honeycutt, the founder of the advocacy organization Moms Across America, found glyphosate contamination in several samples of human breast milk, although the agrochemical industry argued that her results were “implausible.”23
An in vitro study of cells from the gut of both humans and mice by Gildea and co-authors, published in 2017, showed that glyphosate sharply upregulates expression of a protein called zonulin in the gut, which has a powerful ability to induce a leaky gut barrier.24Furthermore, zonulin gets released from a leaky gut barrier into the general circulation, and it then has the ability to also induce a leaky brain barrier.25
Overuse of antibiotics has been blamed for the problems we are now encountering in hospitals with untreatable multiple-antibiotic-resistance pathogenic infections. However, a study published in 2021 has shown that herbicide exposure (including exposure to glyphosate, glufosinate and dicamba) increases the levels of antibiotic resistance genes in diverse soil microbes.26 This likely translates to a similar effect on pathogens that inhabit the gut. This could also help explain why the prevalence of death from intestinal infections in hospital-discharge data is rising in tandem with the rise in glyphosate usage on core crops (see Figure 3).20
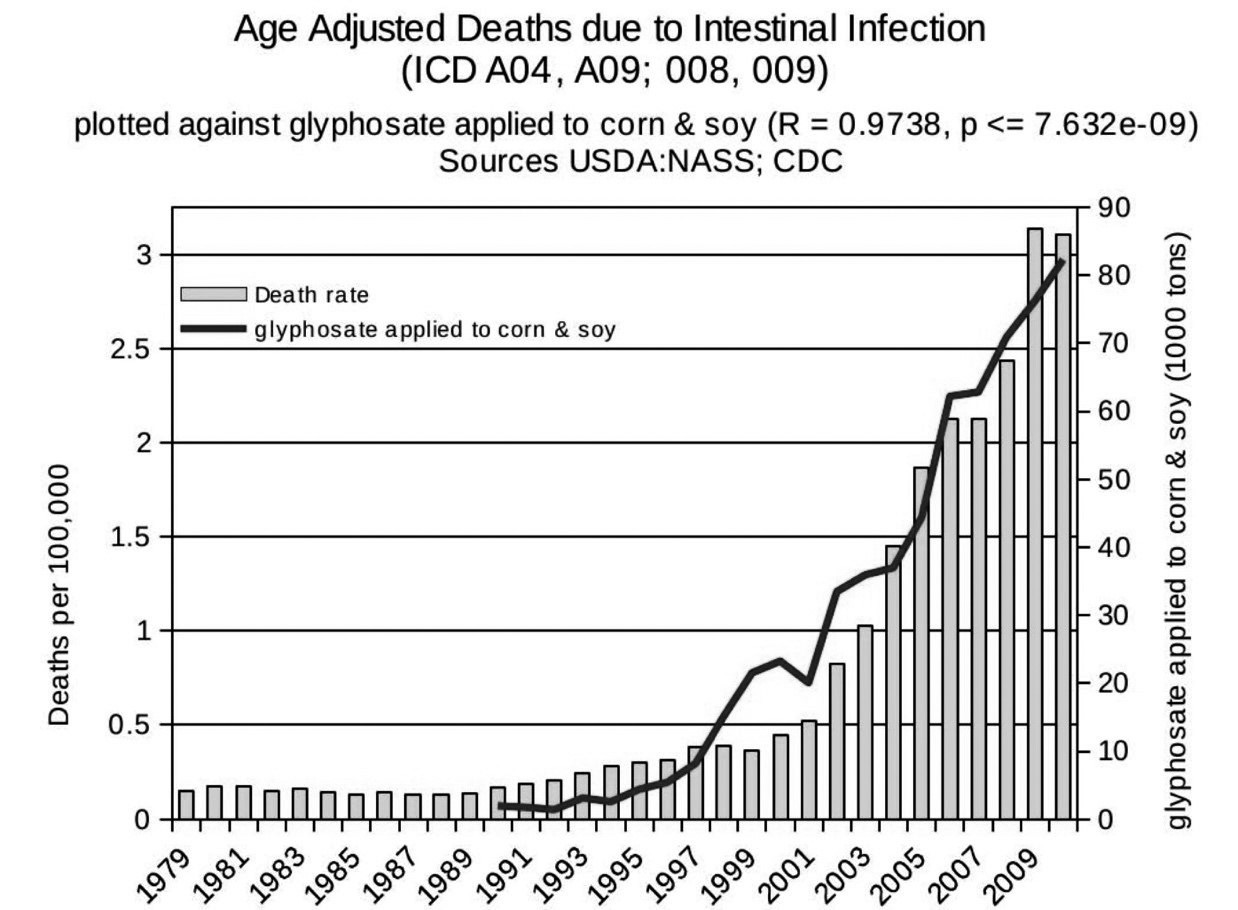
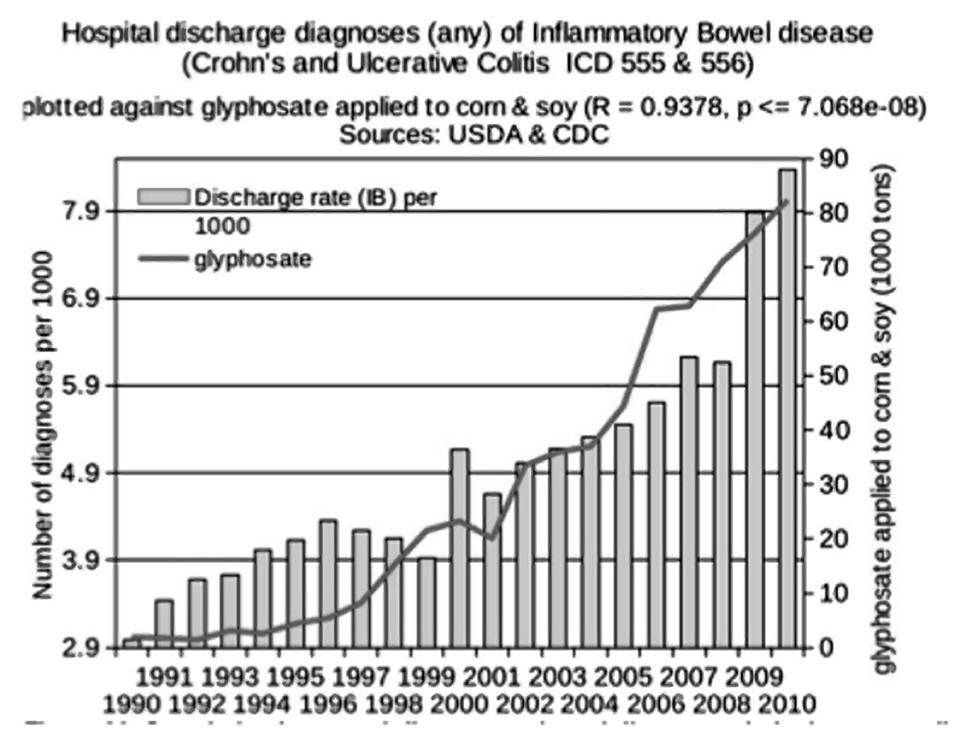
DIGESTIVE ENZYMES, AMMONIA AND COLONIC pH
Even as far back as the 1970s, researchers were aware that acid-loving species such as lactobacillus and bifidobacteria are important to maintain a healthy gut. Vince and co-authors wrote in 1973: “Increased growth of acidophilic organisms such as lactobacillus and bifidobacteria could depress the growth of putrefactive, ammonia-producing organisms such as E. coli and Bacteroides spp.”27 Lactobacillus and bifidobacteria are the two classes of bacteria that are most sensitive to glyphosate.22
Gluten in wheat and casein in dairy foods are both difficult proteins to digest because of their high proline content. Many species of lactobacillus have multiple enzymes that specialize in breaking down proline-rich peptides, so they assist the host in metabolizing these proteins.28 When lactobacillus species are harmed by glyphosate, these peptides are more likely to remain undigested, leading to the epidemic we see today in gluten and casein intolerance.
A study on rats exposed to different levels of glyphosate over a two-week period revealed a very strong correlation between the pH of the feces and the amount of glyphosate detected in the colon (R = 0.72, p < 0.0001).29 The investigators also found a strong inverse correlation between the amount of acetate in the caecum (the first part of the large intestine) and the amount of glyphosate in the caecum (R = 0.54, p < 0.0001). They proposed that glyphosate interfered with the production of acetate by the gut microbes and that this was likely a major contributor to the high pH of the feces.
The colonic gut microbes break down complex carbohydrates that escape digestion in the upper intestine. These carbohydrates may be familiar to you as “prebiotics,” but also as “roughage” and “fiber.” Gut microbes convert them into short-chain fatty acids, primarily acetate, propionate and butyrate. As shown in Figure 4, acetate is the smallest fatty acid, with only a single carbon in the chain (CH3) besides the COOH unit at the end. Propionate adds a second carbon to the chain, and butyrate has three carbons besides the COOH unit.
FATTY ACID BALANCE
The balance among these three, which has important implications for gut health, is strongly influenced by the pH of the gut.30 In particular, butyrate is extremely important for maintaining a healthy gut barrier. The colonocytes lining the wall of the colon are unusual in that their main source of fuel is normally butyrate rather than glucose. In fact, studies have shown that butyrate promotes the development of the intestinal barrier (protects from a leaky gut) by activating a signaling molecule called AMP-activated protein kinase.31
On the other hand, researchers have been able to induce autism-like behaviors in mice by exposing them to high levels of propionate.32 Direct infusion of propionic acid into the brain ventricles of rats caused an inflammatory response associated with an increase in the number of immune cells (microglia) in the brain, as well as immune cell activation.33 This matches the observed features of the human autistic brain. The combination of an excessive number of microglia due to overabundance of propionate combined with a leaky brain barrier due to glyphosate-induced zonulin is a potent formula for brain inflammation. This also demonstrates the powerful capabilities of the gut-brain axis in linking gut problems to neurological diseases.
An in vitro study of the growth of gut bacteria under controlled pH conditions revealed a remarkable pattern that is consistent with glyphosate’s effects on microbial distributions and on pH.34 A pH of 5.5 strongly favors butyrate production, which was four-fold higher at pH 5.5 than at pH 6.5. Propionate increased by two-fold under the higher pH conditions. These changes corresponded to a remarkable increase in bacteroides at the higher pH, coming to represent on average 78 percent of the total eubacteria present. This is especially interesting in line with the observation that Crohn’s disease (an inflammatory bowel disease) is associated with an increase in bacteroides and a decrease in butyrate in the colon.35 Symptoms of Crohn’s include abdominal pain, severe diarrhea, fatigue, weight loss and malnutrition. The effect of pH on butyrate concentration was significant at the 0.001 percent level.34Supplying more peptides led to an increase in propionate synthesis, which was also significant at the 0.001 percent level.
One logical reason for a high pH following chronic glyphosate exposure could be the disruption of protein degradation caused by glyphosate infiltration into digestive enzymes. As reported in our sixth paper together,36 Anthony Samsel and I found remarkably high levels of glyphosate contamination in the digestive enzymes trypsin, pepsin and lipase derived from pigs, which he obtained from a chemistry lab. It is logical that glyphosate is disrupting the ability of trypsin and pepsin to digest proteins, as well as the ability of lipase to digest fats. Undigested proteins would make their way into the colon as peptides (protein fragments), where they would be broken down by the gut microbiome, releasing ammonia.37Ammonia has a very high pKa, which means that it would drive the colonic pH up. Acetate (acetic acid), as its name implies, is acidic, so it would lower the pH, but it is reduced by the influence of glyphosate.
A TALE OF TWO COUNTRIES
A study published in 2016 made an astute observation: “A microcosm of the global gradient in immune disease incidence occurs at the border between Finland and Russian Karelia, where there is a 2- to 6-fold higher incidence of allergies and a 5- to 6-fold higher incidence of T1D [type 1 diabetes] and other autoimmune disorders in Finland relative to Russian Karelia.”38
I suspect that this difference reflects differing degrees of exposure to glyphosate in the two regions. Finnish children are more exposed to glyphosate than their Russian counterparts on the other side of the border, and they have been for years. The use of glyphosate in agriculture, particularly cereal crops, has increased significantly in Finland since 1999. Since 2001, the Finnish government has subsidized farmers for no-till agricultural practices, which use glyphosate to clear the weeds rather than digging up the soil, in order to minimize phosphate run-off into marine waters.39
A study of Finnish children at high risk of developing type 1 diabetes revealed that a clear risk factor was the overgrowth of Bacteroides dorei in children who later became diabetic, which began when solid foods were first introduced.40 Another study, which directly compared the Finnish children with the Russian ones, found that only 10 percent of the Finnish infants harboured Bifidobacterium longum infantis, whereas Russian infants commonly maintained this strain at high levels in infancy.38 In a follow-on study published in 2019, these same authors noted a geographical trend of increasing levels of B. infantis among feces in children from Finland versus Estonia versus Russia.41 They suggested that elevated fecal pH associated with loss of bifidobacteria would promote “inflammation-favoring bacteria and gut dysbiosis.” These differences could be a consequence of higher exposure to glyphosate in the Finnish children, due to greater consumption of glyphosate-contaminated processed foods characteristic of a Western diet.

YEAST OVERGROWTH AND AUTO-BREWERY SYNDROME
A fascinating paper, published in 2016, presented a case study of a seventy-one-year-old man suffering from a long history of Crohn’s disease. It provides a good model for a kind of “worst-case” scenario for escalating gut issues likely caused by chronic exposure to glyphosate year by year over decades.42 The man was surgically treated for rectal cancer in 1987 and began to develop recurrent bowel obstructions in 2012, associated with active Crohn’s inflammation throughout the proximal small bowel. Diarrhea and bloating were found to be caused by small intestinal bacterial overgrowth (SIBO). Throughout 2013 and 2014, he took repeated courses of antibiotics to keep the bacterial overgrowth in check. However, he continued to lose weight and decided to proactively increase his sugar intake in an attempt to stop the weight loss. He started drinking six to eight sodas a day, as well as eating many sugary snacks. In March of 2014, recurrent diarrhea prompted him to resume antibiotic treatments.
It was in this context that he began to notice foggy thinking and difficulty with walking. His wife noticed slurred speech, and he fell while showering. Upon admission to the emergency room (ER), nothing untoward was noted, and he was sent home. However, symptoms continued to worsen, and the next day he returned to the ER. This time, it was found that his blood ethanol level was 234 mg/dL. (For a point of reference, greater than 80 mg/dL is considered “driving under the influence,” and greater than 300-400 mg/dL is considered potentially fatal.) His wife vehemently agreed when he denied having consumed any alcohol for over thirty years.
The explanation for this surprising scenario is that sugar was being fermented to alcohol by resident yeast massively colonizing his gastrointestinal tract. Such a phenomenon has a name: “endogenous ethanol fermentation.” It is also called, more imaginatively, “auto-brewery syndrome.” All the antibiotics the man was taking had killed off enough bacteria to permit an overgrowth of yeast, particularly candida, fueled by the high sugar intake. Switching to a low-carb diet greatly ameliorated the situation.
Inflammation promotes colonization by candida, and, furthermore, candida delays healing of inflammatory lesions, in a vicious cycle. Various studies have reported that a large percentage of patients suffering from Crohn’s disease, ulcerative colitis and gastric and duodenal ulcers—ranging from 37 percent to 86 percent—have an overgrowth of candida in their gut.43
Auto-brewery syndrome causes a lot of uncomfortable symptoms, including belching, chronic fatigue, dizziness, dry mouth, disorientation, hangovers and irritable bowel syndrome. It can also lead to anxiety, depression and reduced productivity.44 Both diabetes and liver cirrhosis lead to a substantially increased production of ethanol in the gut following high carbohydrate ingestion, and the presence of both diseases shows approximately a three-fold increase compared to either disease alone.45 Both diabetes and liver disease are increasing in prevalence in step with the rise in glyphosate usage.20 Furthermore, many studies have shown that glyphosate causes inflammation and fatty liver disease in rats exposed to levels below regulatory limits.46
Auto-brewery syndrome arises due to the confluence of at least three factors. A shortened or incompetent bowel causes impaired nutrient uptake—such that nutrients linger in the gut, supporting the growth of yeast—and repeated antibacterial treatments lead to a reduction in bacterial species, which provides opportunistic space for yeast. Furthermore, liver disease can cause an impaired ability to metabolize alcohol, so the ethanol produced by yeast remains in the bloodstream much longer, accumulating there.
We have an epidemic today in candidiasis, an overgrowth of candida species in the gut and elsewhere in the body.47 We have witnessed a dramatic increase in multiple-cause mortality due to fungal infections, from one thousand five hundred fifty-seven deaths in 1980 to six thousand five hundred thirty-four deaths in 1997.48 Not only candida but also aspergillus and cryptococcus (other fungal species) were involved.
Fungus infection can lead to sepsis, a life-threatening blood disease. The number of cases of sepsis caused by fungi in the United States increased by 207 percent between 1979 and 2000.49 I am guessing that there has been a continued increase since 2000, but I have been unable to locate a paper that provides statistics on fungal infection during the 2000s.
THE AUTISM GUT AND GUT PATHOGENS
BTBR mice are a special breed of mice that were created through selection of the most autistic-like offspring through multiple generations of mice grown in the laboratory. They now breed true to an autistic-like phenotype.
Many studies have been conducted on these mice, some of which involve a detailed characterization of disruptions in their guts. A paper published in 2017 looked specifically at gastrointestinal dysfunction in these mice, with several findings that are consistent with what I would predict from chronic glyphosate poisoning.50 In particular, these mice suffered from a deficiency in bile acid synthesis, which could be due to cytochrome P450 enzyme suppression by glyphosate.51 They also exhibited serotonin deficiency, impaired peristalsis (slowed gut motility), SIBO and a reduction in acetate levels in the gut.
Other studies have shown that BTBR mice have insufficient heparan sulfate in their brain ventricles52 and low plasma concentrations of sulfate.53 In postmortem studies, researchers have also found that autistic children have low heparan sulfate in their brain ventricles.54 As well, children with autism have low plasma sulfate along with high levels of urinary sulfate, reflecting a flushing of sulfate into the urine.55 This could be due to the need to conjugate toxic phenolic compounds produced by gut pathogens with sulfate in order to solubilize them for excretion, as I mentioned earlier. In a recently published paper, my colleague Greg Nigh and I showed how glyphosate could be expected to cause impaired sulfate homeostasis, through multiple mechanisms.56
A study published in 2013 examined the levels of free amino acids in the feces of three groups of children: autistic children, children suffering from pervasive developmental disorder-not otherwise specified (PDD-NOS) and normal controls.57 They found significantly higher levels of most of the amino acids in the feces of autistic children compared to normal controls; the PDD-NOS children also had elevated levels, although less dramatically so. This suggests to me that protein metabolism by trypsin and pepsin is impaired, and peptides are then broken down into free amino acids by the colonic microbes and ultimately excreted in the feces. Unfortunately, the aromatic amino acids, in particular, can be transformed into a variety of different toxic phenolic compounds by several strains of clostridia. Such compounds— such as p-cresol, 4EPS, 4-cresol HPHPA (3-(3-hydroxyphenyl)-3-hydroxypropionic acid) and 4-HPA (4-hydroxyphenylacetate)—can disrupt the function of the neurotransmitters that are also derived from aromatic amino acids.
A review paper published in 2018 hypothesized that glyphosate could play a causal role in autism through its disruption of the gut microbiome; specifically, through the increase in clostridia species inhabiting the gut in response to chronic glyphosate exposure.58The authors hypothesized that toxic metabolites produced by overabundant strains of clostridia could be disrupting neurite outgrowth in the brain. They made the interesting observation that a woman who attempted suicide through glyphosate ingestion was found to suffer subsequently from Clostridium tertium bacteremia.59 Another paper published in 2019 also suggested that glyphosate’s disruption of the gut microbiome could induce an overgrowth of both clostridia and salmonella species; the authors emphasized the neurotoxicity of certain clostridia metabolites.60
A very disturbing paper by William Shaw involved a case study of triplets who appeared to have been damaged by glyphosate exposure.61 The two boys were both diagnosed with autism, and the girl had a seizure disorder. All three were found to have high levels of glyphosate in their urine. The boys also tested high for urinary levels of HPHPA, 4-cresol and 4-HPA. The mother said that non-organic corn tortillas were a major part of their diet. A switch to an organic diet led to a decrease in urinary glyphosate. Another urinary marker, succinic acid, was elevated in the urine in all three triplets. This is an indicator of mitochondrial dysfunction, which has been linked to glyphosate.62
These phenolic compounds synthesized by various clostridia species interfere with dopamine-to-epinephrine metabolism in the brain by the enzyme dopamine β hydroxylase. This leads to excessive dopamine in the brain, along with deficiencies in epinephrine.61 Symptoms of excess dopamine include obsessive, compulsive and stereotypical behaviors, whereas low epinephrine is associated with reduced exploratory behavior and impaired learning in novel environments. An increase in dopamine can be detected through its metabolite homovanillic acid (HVA), which was found at elevated urinary levels in all three triplets.
CONSTIPATION
Constipation has become a serious problem among Americans. Today, constipation affects from 12 to 19 percent of the U.S. population, and the numbers are alarmingly on the rise. In a data analysis for nearly one thousand U.S. hospitals, the number of ER visits for constipation rose 42 percent from 2006 to 2011.63
It is likely that glyphosate plays a role in the epidemic we are seeing in constipation. A distinct possibility is that glyphosate is interfering with the ability of the contractile protein myosin to contract, essentially paralyzing the gut. A case study involving another woman who attempted to kill herself by ingesting glyphosate was remarkable for the clear evidence of paralytic ileus as a severe reaction to acute glyphosate exposure.64 Paralytic ileus is defined as obstruction of the intestine caused by paralysis of the intestinal muscles. Autism and the rigid compulsive behaviors linked to autism are associated with severe constipation with co-occuring intermittent diarrhea.65
LESSONS FROM WORMS
One of the diseases that Swanson and co-authors identified as rising in prevalence in tandem with glyphosate usage in the United States is Parkinson’s disease. The authors found a correlation coefficient of 0.875 (p ≤ 1.6×10−6) between the rise in deaths attributed to Parkinson’s disease and the rise in glyphosate usage.20 It is now well established that Parkinson’s begins in the gut, where misfolded prion-like proteins appearing first in the gut are transported along the vagus nerve to reach the brainstem nuclei.66 Damage to the substantia nigra where dopamine-synthesizing neurons are concentrated eventually leads to Parkinson’s disease.
In a clever experiment, researchers grew grass and seeds in pots and then placed burrowing earthworms in the pots.67 They applied glyphosate at half the amount recommended for agricultural use and then observed the earthworm behavior, compared to a control group. They were predicting that, because glyphosate would kill the plants, there would be abundant decaying food at the surface of the soil that would inspire the earthworms to come to the surface to feast. However, they found that the exposed earthworms left castings on the surface much less frequently than the control group did. After three weeks, their castings virtually stopped. It is likely that they had developed a Parkinsonian-like syndrome that immobilized them.
This idea gains support from another experiment involving roundworms.68 Glyphosate exposure was found to impair their mobility and to specifically damage dopamine-releasing neurons in the roundworms’ brains. These neurons are analogous to the dopamine-releasing neurons in the substantia nigra of humans suffering from Parkinson’s disease.
A spectacular new study on roundworms was very well designed to address the question of whether the microbes in the gut play a role in neurodenegerative diseases.69 These roundworms were engineered to overexpress a peptide sequence called polyglutamine, which is known to misfold into a toxic fibrillar prion-like protein that could lead to neurodegeneration. The researchers showed first that the roundworms developed impaired mobility when exposed to pathogens normally found in the human gut, most notably Klebsiella pneumoniae and Pseudomonas aeruginosa.
Both of these pathogens present major problems today in hospitals due to multiple antibiotic resistance. However, co-colonization with beneficial strains of bacteria that produce abundant butyrate offset the disease process by reducing the amount of misfolded protein in the gut. Supplementation with butyrate was similarly beneficial. Probably the very best natural source of butyrate is organic butter from grass-fed cows. The fact that butyrate depends on a low gut pH, while glyphosate raises the gut pH, implies that glyphosate would reduce the amount of butyrate produced in the gut, which in turn would increase the rate of Parkinson’s disease.
CONCLUSION
The human gut is a remarkable organ-system where human cells collaborate with myriad and diverse gut microbes to perform multiple essential tasks assuring an adequate supply of nutrients to the body. However, when the gut is exposed daily to a toxic poison like glyphosate, many aspects of this elegant system break down. A cascading effect occurs when proteins are not properly broken down, and when pathogens outcompete beneficial species, which are more susceptible to glyphosate’s damaging effects. A summary of the damaging effects of a high-sugar, high-protein, inorganic diet is shown in Figure 5.
An imbalance and/or a deficiency in three short-chain fatty acids—acetate, propionate and butyrate—has broad consequences not only on gut health but also on brain health. A seemingly simple thing like a rise in pH can have profound effects. A general deficiency in bacteria can induce an overgrowth of candida, which can be further encouraged by a high-sugar diet. Remarkably, this can cause a person to become drunk without ever drinking alcohol.
Parkinson’s disease is but one of many neurodegenerative diseases whose origins are found in the gut. Studies on worms exposed to glyphosate can teach us a lot about how glyphosate could be causing an epidemic in Parkinson’s disease, which we are experiencing today. Eating a diet that is high in natural organic animal fats, especially butter, will protect you against Parkinson’s disease. More generally, switching to a certified organic whole foods diet is a very simple and effective strategy for maintaining a healthy gut and a healthy brain.
FURTHER READING - ALTERNATIVES TO GLYPHOSATE PESTICIDES
Since sharing the 'Glyphosate on the Gut' article from on the Weston A. Price Foundation website, we've been contacted by Drugwatch: a free web resource committed to ensuring the safety of families and providing vital health information.
They have created a guide that offers organic, homemade, and agricultural alternatives to mitigate the risks associated with glyphosate. Please feel free to explore them:
www.drugwatch.com/roundup/
www.drugwatch.com/roundup/alternatives/
BROWSE MICROBIOME & GUT HEALTH PRODUCTS
VIEW ALL MICROBIOME & GUT HEALTH PRODUCTS
REFERENCES
- Sender R, Fuchs S, Milo R. Revised estimates for the number of human and bacteria cells in the body. PLoS Biol. 2016;14(8):e1002533.
- Lozupone CA, Stombaugh JI, Gordon JI, et al. Diversity, stability and resilience of the human gut microbiota. Nature. 2012;489(7415):220-230.
- Magnúsdóttir S, Ravcheev D, de Crecy-Lagard V, Thiele I. Systematic genome assessment of B-vitamin biosynthesis suggests co-operation among gut microbes. Front Genet. 2015;6:148.
- Degnan PH, Taga ME, Goodman AL. Vitamin B12 as a modulator of gut microbial ecology. Cell Metab. 2014;20(5):769-778.
- Sudo N, Chida Y, Aiba Y, et al. Postnatal microbial colonization programs the hypothalamic-pituitary-adrenal system for stress response in mice. J Physiol. 2004;558(Pt 1):263-275.
- Martin CR, Osadchiy V, Kalani A, Mayer EA. The brain-gut-microbiome axis. Cell Mol Gastroenterol Hepatol. 2018;6(2):133-148.
- Cristiano C, Lama A, Lembo F, et al. Interplay between peripheral and central inflammation in autism spectrum disorders: possible nutritional and therapeutic strategies. Front Physiol. 2018;9:184.
- Tran SMS, Mohajeri MH. The role of gut bacterial metabolites in brain development, aging and disease. Nutrients. 2021;13(3):732.
- Möhle L, Mattei D, Heimesaat MM, et al. Ly6C(hi) monocytes provide a link between antibiotic-induced changes in gut microbiota and adult hippocampal neurogenesis. Cell Rep. 2016;15(9):1945-1956.
- Manohar H, Pravallika M, Kandasamy P, et al. Role of exclusive breastfeeding in conferring protection in children at-risk for autism spectrum disorder: results from a sibling case-control study. J Neurosci Rural Pract. 2019;9(1):132-136.
- Hsiao EY, McBride SW, Hsien S, et al. Microbiota modulate behavioral and physiological abnormalities associated with neurodevelopmental disorders. Cell. 2013;155(7):1451-1463.
- Persico AM, Napolioni V. Urinary p-cresol in autism spectrum disorder. Neurotoxicol Teratol. 2013;36:82-90.
- Schultz ST, Gould GG. Acetaminophen use for fever in children associated with autism spectrum disorder. Autism Open Access. 2016;6(2):170.
- Ji Y, Azuine RE, Zhang Y, et al. Association of cord plasma biomarkers of in utero acetaminophen exposure with risk of attention-deficit/hyperactivity disorder and autism spectrum disorder in childhood. JAMA Psychiatry. 2020;77(2):180-189.
- Lai SK, Wang YY, Wirtz D, Hanes J. Micro- and macrorheology of mucus. Adv Drug Deliv Rev. 2009;61(2):86-100.
- Rho JH, Wright DP, Christie DL, et al. A novel mechanism for desulfation of mucin: identification and cloning of a mucin-desulfating glycosidase (sulfoglycosidase) from Prevotella strain RS2. J Bacteriol. 2005;187(5):1543-1551.
- Henrick BM, Hutton AA, Palumbo MC, et al. Elevated fecal pH indicates a profound change in the breast-fed infant gut microbiome due to reduction of Bifidobacterium over the past century. mSphere. 2018;3(2):e00041-18.
- Logan WR. The intestinal flora of infants and young children. J Pathol Bacteriol. 1913;18:527-551.
- Frese SA, Hutton AA, Contreras LN, et al. Persistence of supplemented Bifidobacterium longum subsp. infantis EVC001 in breastfed infants. mSphere. 2017;2(6):e00501-17.
- Swanson NL, Leu A, Abrahamson J, Wallet B. Genetically engineered crops, glyphosate and the deterioration of health in the United States of America. Journal of Organic Systems. 2014;9(2):6-37.
- Leino L, Tall T, Helander M, et al. Classification of the glyphosate target enzyme (5-enolpyruvylshikimate-3-phosphate synthase) for assessing sensitivity of organisms to the herbicide. J Hazard Mater. 2021;408:124556.
- Shehata AA, Schrödl W, Aldin AA, et al. The effect of glyphosate on potential pathogens and beneficial members of poultry microbiota in vitro. Curr Microbiol. 2013;66(4):350-358.
- Bus JS. Analysis of Moms Across America report suggesting bioaccumulation of glyphosate in U.S. mother’s breast milk: implausibility based on inconsistency with available body of glyphosate animal toxicokinetic, human biomonitoring, and physico-chemical data. Regul Toxicol Pharmacol. 2015;73(3):758-764.
- Gildea JJ, Roberts DA, Bush Z. Protective effects of lignite extract supplement on intestinal barrier function in glyphosate-mediated tight junction injury. Journal of Clinical Nutrition & Dietetics. 2017;3(1):1.
- Rahman MT, Ghosh C, Hossain M, et al. IFN-γ, IL- 17A, or zonulin rapidly increase the permeability of the blood-brain and small intestinal epithelial barriers: relevance for neuro-inflammatory diseases. Biochem Biophys Res Commun. 2018;507(1-4):274-279.
- Liao H, Li X, Yang Q, et al. Herbicide selection promotes antibiotic resistance in soil microbiomes. Mol Biol Evol. 2021;38(6):2337-2350.
- Vince A, Dawson AM, Park N, O’Grady F. Ammonia production by intestinal bacteria. Gut. 1973;14(3):171- 177.
- Francavilla R, De Angelis M, Rizzello CG, et al. Selected probiotic lactobacilli have the capacity to hydrolyze gluten peptides during simulated gastrointestinal digestion. Appl Environ Microbiol. 2017;83(14):e00376-17.
- Nielsen LN, Roager HM, Casas ME, et al. Glyphosate has limited short-term effects on commensal bacterial community composition in the gut environment due to sufficient aromatic amino acid levels. Environ Pollut. 2018;233:364-376.
- Ríos-Covián D, Ruas-Madiedo P, Margolles A, et al. Intestinal short chain fatty acids and their link with diet and human health. Front Microbiol. 2016;7:185.
- Peng L, Li ZR, Green RS, et al. Butyrate enhances the intestinal barrier by facilitating tight junction assembly via activation of AMP-activated protein kinase in Caco-2 cell monolayers. J Nutr. 2009;139(9):1619-1625.
- Choi J, Lee S, Won J, et al. Pathophysiological and neurobehavioral characteristics of a propionic acid-mediated autism-like rat model. PLoS One. 2018;13(2):e0192925.
- MacFabe DF, Rodríguez-Capote K, Hoffman JE, et al. A novel rodent model of autism: intraventricular infusions of propionic acid increase locomotor activity and induce neuroinflammation and oxidative stress in discrete regions of adult rat brain. Am J Biochem Biotechnol. 2008;4(2):146-166.
- Walker AW, Duncan SH, McWilliam Leitch EC, et al. pH and peptide supply can radically alter bacterial populations and short-chain fatty acid ratios within microbial communities from the human colon. Appl Environ Microbiol. 2005;71(7):3692-3700.
- Mangin I, Bonnet R, Seksik P, et al. Molecular inventory of faecal microflora in patients with Crohn’s disease. FEMS Microbiol Ecol. 2004;50(1):25-36.
- Samsel A, Seneff S. Glyphosate pathways to modern diseases VI: prions, amyloidoses and autoimmune neurological diseases. Journal of Biological Physics and Chemistry. 2017;17:8-32.
- Newmark HL, Lupton JR. Determinants and consequences of colonic luminal pH: implications for colon cancer. Nutr Cancer. 1990;14(3-4):161-173.
- Vatanen T, Kostic AD, d’Hennezel E, et al. Variation in microbiome LPS immunogenicity contributes to autoimmunity in humans. Cell. 2016;165(4):842-853.
- Salonen J, Hyvönen T, Kaseva J, Jalli H. Impact of changed cropping practices on weed occurrence in spring cereals in Finland – a comparison of surveys in 1997-1999 and 2007-2009. Weed Research. 2013;53(2):110-120.
- Davis-Richardson AG, Ardissone AN, Dias R, et al. Bacteroides dorei dominates gut microbiome prior to autoimmunity in Finnish children at high risk for type 1 diabetes. Front Microbiol. 2014;5:678.
- Vatanen T, Plichta DR, Somani J, et al. Genomic variation and strain-specific functional adaptation in the human gut microbiome during early life. Nat Microbiol. 2019;4(3):470-479.
- Welch BT, Coelho Prabhu N, Walkoff L, Trenkner SW. Auto-brewery syndrome in the setting of long-standing Crohn’s disease: a case report and review of the literature. J Crohns Colitis. 2016;10(12):1448-1450.
- Kumamoto CA. Inflammation and gastrointestinal candida colonization. Curr Opin Microbiol. 2011;14(4):386-391.
- Painter K, Cordell BJ, Sticco KL. Auto-brewery Syndrome. Treasure Island, FL: StatPearls Publishing; 2021 Jan. 2020 Oct 14.
- Hafez EM, Hamad MA, Fouad M, Abdel-Lateff A. Auto-brewery syndrome: ethanol pseudo-toxicity in diabetic and hepatic patients. Hum Exp Toxicol. 2017;36(5):445-450.
- Pandey A, Dhabade P, Kumarasamy A. Inflammatory effects of subacute exposure of Roundup in rat liver and adipose tissue. Dose Response. 2019;17(2):1559325819843380.
- Pfaller MA, Diekema DJ. Epidemiology of invasive candidiasis: a persistent public health problem. Clin Microbiol Rev. 2007;20(1):133-163.
- McNeil MM, Nash SL, Hajjeh RA, et al. Trends in mortality due to invasive mycotic diseases in the United States, 1980-1997. Clin Infect Dis. 2001;33(5):641-647.
- Martin GS, Mannino DM, Eaton S, et al. The epidemiology of sepsis in the United States from 1979 through 2000. N Engl J Med. 2003;348(16):1546-1554.
- Golubeva AV, Joyce SA, Moloney J, et al. Microbiota-related changes in bile acid & tryptophan metabolism are associated with gastrointestinal dysfunction in a mouse model of autism. EBioMedicine. 2017;24:166-178.
- Hietanen E, Linnainmaa K, Vainio H. Effects of phenoxyherbicides and glyphosate on the hepatic and intestinal biotransformation activities in the rat. Acta Pharmacol Toxicol (Copenh). 1983;53(2):103-112.
- Mercier F, Kwon YC, Douet V. Hippocampus/amygdala alterations, loss of heparan sulfates, fractones and ventricle wall reduction in adult BTBR T+ tf/J mice, animal model for autism. Neurosci Lett. 2012;506(2):208-213.
- Corley MJ, Meyza KZ, Blanchard DC, Blanchard RJ. Reduced sulfate plasma concentrations in the BTBR T+tf/J mouse model of autism. Physiol Behav. 2012;107(5):663-665.
- Pearson BL, Corley MJ, Vasconcellos A, et al. Heparan sulfate deficiency in autistic postmortem brain tissue from the subventricular zone of the lateral ventricles. Behav Brain Res. 2013;243:138-145.
- Waring RH, Klovrza LV. Sulphur metabolism in autism. Journal of Nutritional & Environmental Medicine. 2000;10(1):25-32.
- Seneff S, Nigh G. Sulfate’s critical role for maintaining exclusion zone water: dietary factors leading to deficiencies. Water. 2019;11:22-42.
- De Angelis M, Piccolo M, Vannini L, et al. Fecal microbiota and metabolome of children with autism and pervasive developmental disorder not otherwise specified. PLoS One. 2013;8(10):e76993.
- Argou-Cardozo I, Zeidán-Chuliá F. Clostridium bacteria and autism spectrum conditions: a systematic review and hypothetical contribution of environmental glyphosate levels. Med Sci (Basel). 2018;6(2):29.
- You MJ, Shin GW, Lee CS. Clostridium tertium bacteremia in a patient with glyphosate ingestion. Am J Case Rep. 2015;16:4-7.
- Rueda-Ruzafa L, Cruz F, Roman P, Cardona D. Gut microbiota and neurological effects of glyphosate. Neurotoxicology. 2019;75:1-8.
- Shaw W. Elevated urinary glyphosate and Clostridia metabolites with altered dopamine metabolism in triplets with autistic spectrum disorder or suspected seizure disorder: a case study. Integr Med (Encinitas). 2017;16(1):50-57.
- Pereira AG, Jaramillo ML, Remor AP, et al. Low-concentration exposure to glyphosate-based herbicide modulates the complexes of the mitochondrial respiratory chain and induces mitochondrial hyperpolarization in the Danio rerio brain. Chemosphere. 2018;209:353-362.
- Sommers T, Corban C, Sengupta N, et al. Emergency department burden of constipation in the United States from 2006 to 2011. Am J Gastroenterol. 2015;110(4):572-579.
- Nakae H, Kusanagi M, Okuyama M, Igarashi T. Paralytic ileus induced by glyphosate intoxication successfully treated using Kampo medicine. Acute Med Surg. 2014;2(3):214-218.
- Peters B, Williams KC, Gorrindo P, et al. Rigid-compulsive behaviors are associated with mixed bowel symptoms in autism spectrum disorder. J Autism Dev Disord. 2014;44(6):1425-1432.
- Kim S, Kwon SH, Kam TI, et al. Transneuronal propagation of pathologic α-synuclein from the gut to the brain models Parkinson’s disease. Neuron. 2019;103(4):627-641.e7.
- Gaupp-Berghausen M, Hofer M, Rewald B, Zaller JG. Glyphosate-based herbicides reduce the activity and reproduction of earthworms and lead to increased soil nutrient concentrations. Sci Rep. 2015;5:12886.
- Negga R, Stuart JA, Machen ML, et al. Exposure to glyphosate- and/or Mn/Zn-ethylene-bis-dithiocarbamate-containing pesticides leads to degeneration of γ-aminobutyric acid and dopamine neurons in Caenorhabditis elegans. Neurotox Res. 2012;21(3):281-290.
- Walker AC, Bhargava R, Vaziriyan-Sani AS, et al. Colonization of the Caenorhabditis elegans gut with human enteric bacterial pathogens leads to proteostasis disruption that is rescued by butyrate. PLoS Pathog. 2021;17(5):e1009510.